Seismic Effect on Residents from 3 MW Wind Turbines
Bakker, H.H.C.,
School of Engineering and Advanced Technology, Massey University,
H.H.Bakker@massey.ac.nz
Bennett, D.J., Kea Petroleum Limited, Wellington NZ 6012
davebennett@xtra.co.nz
Rapley, B., Atkinson & Rapley Consulting, Palmerston North,
arg@paradise.net.nz
Thorne, R., Noise Measurement Services, Brisbane,
bob@noisemeasurement.com.au
Abstract
Residents on a river plain at the foot of the Tararua Ranges, New Zealand, experience ongoing noise problems, including sleep deprivation, thought to emanate from a nearby wind farm in the ranges to the east (closest V90 turbine is 3 km away). The problem is worst when wind is from the eastern quadrant. Installation of 'Hush Glass' only partly alleviated the problem indoors.
Continuous time series recording of seismic noise using a buried L4 geophone and acoustic surface microphone attached to a wall inside the house, was conducted during March 2009. Use of night hours records minimised extraneous noise, and seismic noise from vegetation was also guarded against by analysis of site wind records.
Early analysis of 196s seismic samples identifies noise bursts lasting 10 seconds or more, every minute or so, associated with easterly wind conditions; with broad spectral power peaks centred on approximately 10 and 28 Hz. Audio playback of the seismic records was identified by the residents as similar to the noise they experienced.
We conclude that seismic energy from the turbines, most likely as Rayleigh waves, is coupled through its concrete foundations into the house, where various vibrational modes are stimulated, thus producing the effects experienced. We note that residents experience these strongest when lying down, i.e. when best aurally coupled to the foundations.
These results provide an initial indication that seismic effects should be assessed in consideration of offset distances from turbines to residences.
Ongoing work will consider such factors as directionality of seismic noise, proximity to the range front fault as possibly accentuating seismic response, either through standing wave or dispersion, and constructive/destructive interference between turbines associated with wind variability as a cause of the intermittent nature of the phenomenon.
Introduction
The Tararua Ranges in New Zealand provides some of the best wind farm sites in the world with generation factors of over 40%. Several windfarms have recently been constructed and several more are in the planning stages. One of these will be located within ten kilometres of the centre of the city of Palmerston North (pop 75,000).
While much has been published on the acoustic emanations from wind farms (Hayes McKenzie Partnership Ltd (2006), Pedersen & Persson-Waye (2004), van den Berg (2006)) little work has been carried out on vibration. This paper studies the case of residents on the river plain at the foot of the Tararua Ranges who have experienced noise problems from a wind farm with the closest V90 turbine 2.8 km to the south- east. One of the observations made by the residents was that the noise could be heard "through the pillow," suggesting involvement of seismic, as well as acoustic, effects.
This study was undertaken to investigate two issues:
- whether the noise reported by the residents could be detected and analysed, and
- whether it was related to the nearby windfarm.
Background
The residence in question lies at the edge of a river plain at the base of the Tararua Ranges on a spur. Two wind farms lie to the east through to the southeast at the top of the ranges. The older wind farm consists of 48 Vestas V47’s, commissioned in 1999, and 55 Vestas V47’s which were commissioned in May 2004. The newer windfarm comprises 31 Vestas V90’s with commissioning beginning in 2007 and is still on-going. The closest Vestas V90’s are approximately 2.8 km south-east of the residence. The onset of noise problems coincides with the installation of the newer V90 turbines with noise being experienced by a number of neighbouring properties around the residence which is being monitored.
After initial complaints from the residents the power company installed 'Hush Glass' in the master bedroom (surface microphone location - see Figure 1). This reduced the acoustic noise, however, there are still noise issues.
The residence is of timber and brick facade construction with a concrete slab foundation. It is 52.5 metres long and between 9 to 13.5 metres wide with the long axis pointing towards the wind farms. It is divided into three distinct sections with the house separated from a large workshop and office by a carport. The workshop lies closest to the windfarms.
The residents have observed the following pattern to the noise occurrences:
- The noise is audible only when the wind is coming from the south-east.
- It is most noticeable at night, particularly in the early hours of the morning.
- The noise is 'audible' when lying down, "through the pillow," but is also audible in the main bedroom and in the hallway when more severe.
- It consists of a low rumbling noise reminiscent of a jet landing.
- The residents find some alleviation of the problem by transferring to another bedroom of the house, suggesting that the geometry of the main bedroom and its outlook may be a contributing factor.
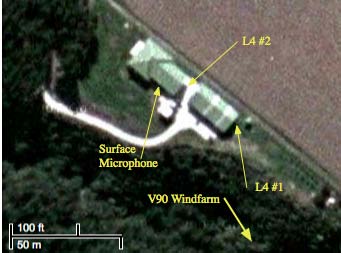
Figure 1: Site layout and position of sensors. (© Copyright 2009 Google Map Data)
Some of the neighbouring residents also observe similar noise issues but report disturbances on different nights, suggesting that the effect is localised.
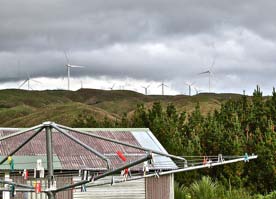
Figure 2: View of the V90 windfarm to the east south-east of the residence.
The Vestas V90 turbine is a 3MW unit with a variable-speed gearbox that allows it to adjust the blade speed to be optimal for the existing wind speed while adjusting blade pitch and the gearbox ratio to smooth the incoming power. The operational speeds range from 0.14Hz to 0.31Hz. Vestas (2008)
Seismic Effects
Ground acceleration associated with earthquakes is a well known phenomenon. In NZ some local authorities produce maps of ground response to illustrate areas of high earthquake hazard. For example, the Wellington Regional Council has such maps on its website, one of which shows that the area immediately west of the Tararua Ranges, just to south of the study area, is predicted to have high ground shaking response to a driving signal from an earthquake. Basically, this is because the geologically ‘young’ and relatively unconsolidated rocks and fill west of the range front, will wobble like jelly when seismically excited; whereas the geologically older and better consolidated rocks of the ranges will have a much smaller response.
In the field of seismic surveying the phenomenon is also well known as ‘ground roll’; an unwanted ‘jelly wobble’ most obvious when working over unconsolidated surface fill such as river gravels or sediments in confined valleys. Seismic companies make routine use of DIN 4150 and similar standards to ensure that ground accelerations at nearby houses, due to the operation of their equipment, fall within acceptable standards since the phenomenon of unwanted vibration and noise within a residence is a common cause of complaint. Rayleigh Waves, or ‘ground roll’, propagate energy along the air-earth boundary, with particle motion confined to the vertical plane in the direction of travel. The energy propagates outwards with a geometrical spreading attenuation factor of 1/(range). This contrasts with propagation of compressional (acoustic) energy through the air which attenuates as 1/(range**2).
Styles (2005) reported on the measurement of seismic vibrations from a wind farm in Scotland. He noted that such seismic signals can travel for tens of kilometres and still be measurable. The seismic vibrations observed were predominantly at the rotational and blade-passing frequencies and their harmonics. In the case of the Vestas V90 turbines this would be 0.14-0.31Hz and 0.43-0.92Hz respectively. However. Styles is careful to comment that these seismic signals are below human perception threshold.
The question, therefore, is not whether turbines actually generate seismic energy, but whether this signal can be significant for nearby residents. In the present instance, the turbines are well coupled into the mechanically competent ‘old’ rocks of the ranges by their concrete bases, which will ensure good coupling of whatever seismic energy they generate into the ground. Rayleigh Wave energy will then propagate outwards, including towards the range front to the west, where they will meet the unconsolidated sedimentary rocks and sediments of the river terraces to west, on which the residents' house is situated. The range front fault system will be a driving point for energy propagation into the sedimentary rocks to the west, rather analogous to shaking a carpet from the edge and sending waves through it. Given the Rayleigh velocity in such rocks, at frequencies of 1-10 Hz typical wavelengths will be hundreds of metres to ~1 km, with standing waves setting up nodal and antinodal points. It is possible that the residents' house, some 2 km west of the range front, is at an antinodal point in certain conditions. The effect may also be exacerbated by the trace of the range front, which effectively runs due north to the north, and southwest to the south of the residents' house. This may serve to focus Rayleigh energy propagating west from the range front into the vicinity of the residents' house, rather like the focal point of a parabolic reflector; and may explain why the unwanted effects are localised to a few neighbouring houses.
Experimental
Initial measurements used two sensors to measure the vibrations in the main bedroom and seismic vibrations in the ground adjoining the house. An acoustic Soho surface microphone was placed on the wall of the main bedroom equidistant from the underlying studs and cross-members. A Mark Products L4 1Hz geophone was buried in the ground at the workshop end of the residence.
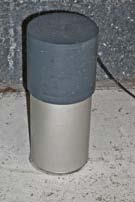
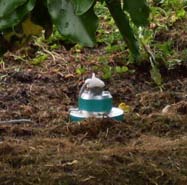
Figure 3: Photo of L4 geophones in situ; bonded to the carport floor and buried in the ground.
Two separate data acquisition units were used to record data. A LabJack U3-HV was used to record two channels at a 100Hz-sampling rate and 10 bits of resolution. Another PC-based instrument, SAM - a spectroaudiometer, (Rapley and Atkinson Consulting, Palmerston North, New Zealand) was used to capture data.
SAM is based on National Instruments' Virtual Instrumentation core and provides the ability to record and analyse sound data, providing real time power spectra as well as statistical data on environmental noise every second, such as the equivalent sound level. It is more commonly used to monitor nuisance noise and is capable of creating a number of different on-the-spot reports including a noise abatement notice (complete with trace of noise level over time and GPS-based location.) It was used for this work for its ability to continuously record data at 100Hz as well as 44.1kHz data captures when set power levels were exceeded.
The standard microphones that come with the equipment were replaced by the surface microphone and L4 geophones. Data was measured at 44.1kHz and 16 bits of resolution and decimated to 100Hz for continuous recording. The full signal was also recorded for 60s after power levels exceeded a set threshold with a hold-off period of 10 or 20 minutes thereafter.
A USB sound card was used to digitise the incoming signals after they had been passed through an Australian Monitor AMIS-PRE1 preamplifier and a low-pass antialiasing filter. The acoustic microphone had an additional preamplifier at the main bedroom to boost the signal before being sent the 30m to the data acquisition systems.
An Acer laptop computer was initially used to run SAM but was later changed to a desktop computer when issues were found with interference from the internal circuitry of the Acer and with its ability to handle the data load. A WS1083 Black Weather Station PC USB was installed at the residence providing half-hourly summaries of wind direction, wind speed, rainfall as well as other sundry data.
Figure 4: Weather station mounted close to the south-eastern end of the residence.
Data was periodically retrieved and analysed using Matlab (The Mathworks, Natick, USA). The data was first presented for rapid visual inspection by plotting three minute periods of time series, power spectrum, wind speed/direction and rainfall data and converting the resulting plots into a movie. (see Figure 5) This provided an effective method of scanning the data rapidly, with frame-by-frame control both backwards and forwards, as well as providing a low data storage method to summarise the data.
Figure 5: One frame of a movie to store a day's worth of data in a readily viewable
form. (For wind direction, 0° is east.)
From these plots events were identified and analysed. Only events that occurred between about 10:30pm and 5am were considered as possible seismic events as the residents were not active during these times. Where possible the 44.1kHz sampled data was used, i.e. when a one-minute sampled interval aligned with the event in question.
Events were then compared with the residents' recollections from the same times, backed up by a diary that they kept of instances of noise. Some events were played back to the residents using either earphones or a stereo system (to provide reasonable playback of the low frequencies in the signals) to help identify the event. Cross-correlations and auto-correlations were taken of the two signals.
Results
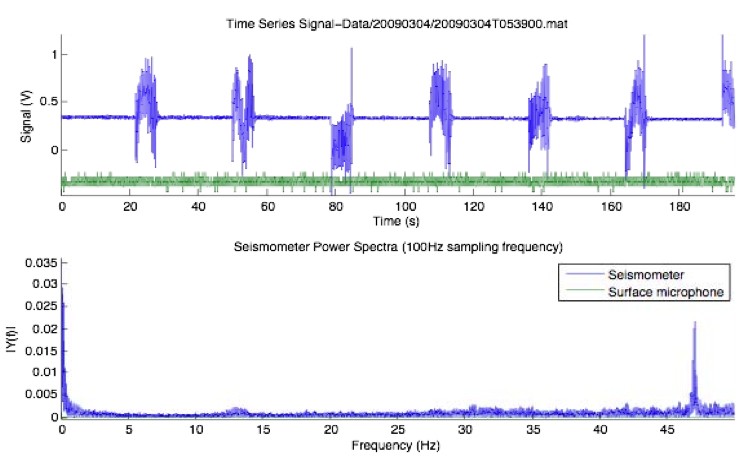
Figure 6: Time series signals and power spectra of domestic water pump.
Figure 7: Time series signals and power spectra during strong winds with an average
speed of 8.8m/s and a peak gust of 12.9m/s.
Figure 8: Time series signals and power spectra of human footsteps in the workshop
and closing of the workshop door (centre).
Figure 9: Time series signals and power spectra of a typical seismic event (recorded
at 2:45am at an average wind speed of 1.4m/s and a peak of 2m/s).
Figure 10: Auto-correlation plots of the time series signals in Figure 9.
Figure 11: Cross-correlation plot of the time series signals in Figure 9.
Figure 12: Time series signals and power spectra of a typical seismic event over a
long baseline taken at an average wind speed of 5m/s with gusts to about 7m/s.
Figure 13: Detail of Figure 12 from 0Hz to 5Hz.
Discussion
Initial results indicated that artefacts were generated at 1Hz and 5Hz for many signals and at 4Hz for the surface microphone signals. These appeared to be due to electrical noise injection from the laptop computer in to the USB sound card, which was replaced for later experiments.
A number of extraneous events were identified and removed from subsequent analysis:
- The water pump supplying domestic water. As shown in Figure 6 this produced tight bursts of high intensity noise in the time domain with a large near-DC content in the power spectrum and a peak at 47Hz. This latter is a strong indicator of an AC motor running, with slip, at slightly less than the 50Hz mains power.
- High wind. As shown in Figure 7, this presumably coupled to the ground through the house itself. This was characterised by many spread impulses of varying amplitudes in the time domain and fairly constant energy from DC to beyond 100 Hz in the frequency domain. Broad peaks at 32Hz, 46Hz are present. Note the artefacts at 1Hz, 5Hz and their harmonics.
- Footsteps in and around the workshop. These provided a rough calibration of the seismometer gain, which was otherwise uncalibrated. Figure 8 shows a typical event with regularly spaced impulses in the time series signal both increasing and decreasing in intensity and associated with a broad peak at 32Hz and smaller peaks at 8Hz and 50Hz in the power spectrum.
After eliminating these events there were a number remaining with similar characteristics that appeared only when the wind was from a south-easterly direction, and reached maximum intensity on those nights when the residents reported the loudest nuisance noise. One of the clearest and strongest events is shown in Figure 9 with sharp and complex bursts in the time series signal generally lasting in the order of 10 seconds and with broad peaks at 28Hz and 10Hz. The intensity of these events was seen to increase with wind speed above about 1m/s and appeared to disappear for wind speeds much above about 6m/s.
The frequencies of 28Hz peak are near the lower threshold of human hearing— normally quoted as 20Hz—but there is significant energy as high as 35-40Hz. This would be perceived as a very low rumble similar to that described by the residents and would be amplified by mechanical coupling. As noted by Moller (2004), at these frequencies the dynamic range of human hearing is markedly decreased and with a spread of individual thresholds. This can lead to large differences in perceived loudness; a sound that is inaudible to one person can be loud to another.
When recordings of these were played back to the residents through either earbuds or a stereo system (good bass response was required to make the low frequencies audible) they reported that the recordings were similar to the noise that they experienced. This represents good evidence that the noise reported by the residents has been detected by the seismometer. In conjunction with the relative success of the 'Hush Glass,' it is possible to conclude that there are two parts to the nuisance noise; an acoustic wave modulated in amplitude, and possibly frequency, and a seismic wave that is 'perceivable' within the residence creating sleep disturbance.
Auto-correlations of the signals were carried out (Figure 10) showing that both signals had a strong non-periodic component although the seismometer signal showed a periodic component at close to 30Hz. The cross-correlation of the two signals (Figure 11) suggests a similar component at close to 30Hz.
One interpretation of these data would be that the broad peaks in the spectrum of Figure 9 were caused by vibrational modes in the windfarm structures. One could postulate either a vibrational resonance mode or multi-turbine constructive interference as sources of these vibrations. If the vibrations were coupled to the ground as Rayleigh waves, they could propagate principally in the upwind and downwind directions from the towers, and therefore only be noticed when the wind was blowing from the south-east or north-west. They would dissipate with distance rather than its square and be measurable at larger distances. In this case the concave shape of the fault front at the base of the range could act as a lens, focussing these vibrations to a small area in which the residence in question, and one or two others, was situated.
From previous experiments, Styles (2005), seismic recording of wind turbines have shown peaks at the rotational and blade-passing frequencies as well as their harmonics. The relevant frequencies for this windfarm are 0.14-0.31Hz and 0.43- 0.92Hz respectively. A long baseline sample was analysed to provide higher definition at these lower frequencies (Figure 12). Apart from several small peaks close to 0.1Hz, there are no significant peaks suggesting either the rotational frequency, blade-passing frequency or any of their harmonics (Figure 13) that might be emitted from the windfarm. As noted in Styles (2005a) these frequencies were at very small levels of vibration and would not normally be detectable with conventional instruments.
An alternative explanation for the seismic events are that the house is not seismically coupled to the windfarm but acoustically. In this case it would be the acoustic noise making the house and concrete pad vibrate thereby causing the seismic vibrations measured by the geophone. This would explain the similarity in the power spectra of the footsteps and the seismic events. Further work needs to be undertaken to differentiate between the competing hypotheses and should consider such factors as directionality of seismic noise, proximity to the range front fault as possibly accentuating seismic response—either through standing wave or dispersion—and constructive/destructive interference between turbines associated with wind variability as a cause of the intermittent nature of the phenomenon.
Conclusions
Seismic and acoustic measurements were undertaken at a residential site at the base of the Tararua Ranges close to a windfarm to determine whether nuisance noise reported by the residents could be detected and whether it could be traced to the windfarm.
Extraneous events were eliminated from the measurements by using only night time records, by removing known events and by eliminating events that did not correlate with the timing of the residents' perception.
The remaining events were characterised by bursts of around 10 seconds duration and with broad peaks in the power spectra at 28Hz and 10Hz.
When the residents were played these events, through earbuds or a stereo system, they decided that they were closely similar to the noise they had been reporting. We therefore conclude that the noise 'perceived' by the residents is measurable, consists of separate acoustic and seismic parts and can cause annoyance by disturbing sleep.
Seismic emanations recorded at other windfarms, Styles (2005), were not observed in the records. Several hypotheses have been suggested for the source of the seismic spectral peaks involving the windfarm but the data available cannot confirm or disprove any of them. Ongoing work will continue to analyse the events and seek to do so.
References
Hayes McKenzie Partnership Ltd (2006) "The measurement of low frequency noise at three UK wind farms", report to DTI, URN number 06/1412
Moller H, Pedersen CS. (2004) "Hearing at low and infrasonic frequencies", Noise Health [serial online] [cited 2009 May 3];6:37-57. Available from: http://www.noiseandhealth.org/text.asp?2004/6/23/37/31664
Pedersen, E & Persson-Waye, K (2004) "Perception and annoyance due to wind turbine noise - a dose - response relationship", Journal of the Acoustical Society of America, 116(6), pp. 3460-3470
Styles, P, Stimpson, I, Toon, S, England, R & Wright, M, (2005) “Microseismic and Infrasound Monitoring of Low Frequency noise and Vibration from Windfarms: Recommendations on the Siting of Windfarms in the Vicinity of Eskdalemuir, Scotland", Report, Keele University, Keele, UK.
Styles, P, Toon, S (2005a) "Wind Farm Noise", Article, British Wind Energy Association, http://www.bwea.com/ref/lfn_keele.html.
van den Berg, GP, (2006) "The sounds of high winds - the effect of atmospheric stability on wind turbine sound and microphone noise", Science Shop for Physics, Netherlands, Electronic version available from: www.ventdecolere.org/archives/nuisances/VAN DEN BERG 2006.pdf
Vestas (2008) 'V90 - 3.0MW An efficient way to more power', Product Brochure, Vestas Wind Systems A/S, Randers, Denmark